Research Areas
Probing Localized Surface Plasmon Resonance (LSPR) Mediated Catalysis Across Multiple Timescales in Emerging Plasmonic Nanomaterials
Plasmon-driven chemistry offers a novel route to chemical transformations and has significant potential impact on applications such as energy conversion and photocatalysis. The processes of plasmon excitation and relaxation, along with the associated cascade of chemical events, occur over multiple time scales. Elucidating the full extent of plasmon-driven chemical events holds a key to deepen our fundamental understanding, and thus enabling novel applications.
​
The goal of this research direction is to study how the electronic states and molecule-plasmon coupling of bulk transition-metal nanoparticles influence surface reactions important to catalysis that include oxygen evolving reactions (OER), hydrogen evolving reactions (HER), water-splitting catalysis, and CO2 reduction. The early ultrafast timescales of plasmon-driven chemistry are not well understood and are potentially research areas conducive to high impact discovery that aids in the design of novel inorganic materials for increased catalytic efficiency.
​
Furthermore, plasmon-driven chemical reactions in earth-abundant emerging plasmonic materials, such as transition-metal nitrides (e.g. titanium nitride TiN), are not yet well understood and the underlying photophysical and photochemical phenomena yield interesting research directions.

Biosensors and microfluidics for the detection of catalytic product formation and small molecule adsorption kinetics (O2, H2, and CO) under quantum confinement.
LSPR-based sensing techniques can be utilized for studying small molecule absorption and binding kinetics relevant for catalysis and soft matter, as well as a diagnostic spectroscopic tool for biomedical applications. This research focus involves utilizing LSPR-based materials including plasmonic nanosphere oligomers and nanosphere lithography thin films to probe dynamic adsorption, binding, and conformational changes in small molecules (i.e. O2, H2, and CO) relevant to catalysis. Extension of this work will also be applied towards studying protein-protein interactions, binding kinetics, and protein conformational changes with increased chemical sensitivity due to LSPR-based biomedical sensing techniques.
​
A microfluidics chamber and flow assembly will be incorporated into an inverted microscope for the development of pump-probe microscopy-based approaches that incorporate a liquid-gas flow cell. Applications of LSPR-based sensing substrates can be utilized for synthesizing new chemical compounds under quantum confinement, for the detection of stimuli-responsive chemical systems, or as a biomedical diagnostic of human health where spectroscopy is utilized to assess substrate accuracy and performance.
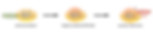
Understanding the photophysical and photochemical dynamics at the electrified solid-liquid interface with surface-enhanced spectroscopies.
Electrochemical technologies are essential for the successful transition to a fossil-free society, but our ability to understand and design is hindered by the many simultaneous processes that occur across orders-of-magnitude differing length scales as these devices operate. Electrochemical devices are inherently complex; when one observes a simple current-voltage relationship, a myriad of phenomena take place which all contribute to the observed current.
​
In situ time-resolved and steady-state surface-enhanced spectroscopies can shed light on these photophysical and photochemical dynamics at the solid-liquid interface, elucidating nuclear rearrangement in chemical reactions coupled with the flow of electrons on ultrafast timescales. Catalytic intermediates and product formation can then be detected in real-time on nanometer length scales.
​
Utilizing localized surface-plasmon resonance (LSPR) based plasmonic materials as a sensing platform, we combine electrochemistry with surface-sensitive spectroscopies, such as surface-enhanced Raman or transient reflectance, for the detection of solid-liquid interfacial behavior such as dynamic adsorption, binding, and conformational changes in molecules relevant to catalysis. These measurements will be coupled with potential-controlled electronic structure methods, allowing first-principles quantification and simulation of the dynamic processes.
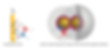